Small-Scale Robotics: From Microbots to Nanobots
The term Small-Scale Robotics is used to refer to those robotic systems whose dimensions can be on the order of nanometers down to millimeters.

In recent years, the term Small-Scale Robotics has been used to refer to those robotic systems whose dimensions can be on the order of nanometers to millimeters. Small-scale robots can be classified into millibots, microbots, and nanobots. Millibots are robotic devices with characteristic dimensions in millimeters.
Microbots have components with dimensions smaller than a millimeter and larger than a micrometer. On the other hand, nanobots have elements with sizes in the order of nanometers and smaller than a micrometer. However, some authors often use the term microbot to refer to robotic devices whose dimensions are tiny compared to conventional robots.
Potential applications for small-scale robots are diverse, including manufacturing, rescue, surveillance, monitoring, defense, and medicine. However, these robots are still considered a scientific curiosity, devices found only in research laboratories or used by a small number of specialized companies.
For these robots to be commercialized, numerous challenges related to their manufacture, control, and power supply must first be overcome to achieve the autonomy that distinguishes any robotic device.
History of small-scale robotics
The origin of small-scale robotics dates back to 1959, when Dr. Richard Feynman, Nobel Laureate in Physics, gave his lecture "There's Plenty of Room at the Bottom". This lecture was an invitation to enter a new field of physics, called nanotechnology. Feynman mentioned the possibility of manipulating, controlling, and constructing objects of small dimensions, establishing the basis for small-scale robots.
With the development of microfabrication technologies for integrated circuits and the emergence of the microcontroller in the 1970s, the means were created to produce ever smaller and more powerful machines. In 1983, Richard Feynman mentioned the possibility of manufacturing small-scale robots and proposed applications for such robots, especially those related to medicine.
In the 1980s, important technological advances were developed, such as the scanning electron microscope and the scanning probe microscope. These advances, along with improved microfabrication techniques, enabled the development of microelectromechanical systems (MEMS), which integrate sensors, motors, and mechanisms for small-scale robots.
In 1988, Anita Flynn proposed the use of a swarm of small, cheap, disposable robots to replace a complex robot. Pister, Katz, and Kahn (1999) proposed the use of multiple wirelessly controlled MEMS devices for sensing parameters such as mechanical vibrations, temperature, magnetic fields, and chemicals. These tiny devices are equipped with everything a small-scale mobile robot needs, except actuation and propulsion.
In 2000, the development of nanoparticle systems designed for drug delivery began. In 2006, the first microscale robots were manufactured using MEMS technology. Currently, small-scale robots capable of implementing sophisticated nanomanipulation sequences and the design of intelligent robot swarms for the military and biomedical industries are under development.
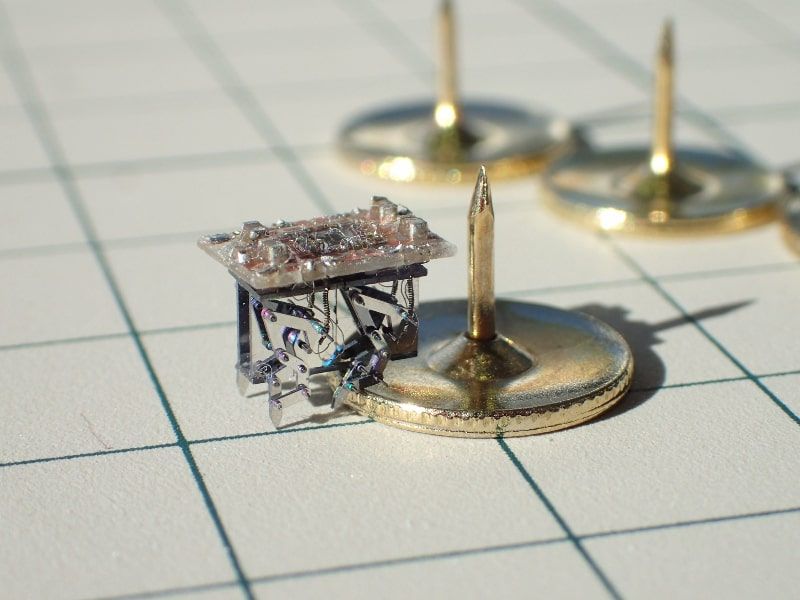
Design of small-scale robotics
The fundamental idea behind the operation of a robot is to mimic the functions of a living being. Living beings collect information from the environment (sensing); this information is processed and the most relevant data is extracted to make a choice (control) and implement it to adjust our behavior (actuation). For the implementation of the above functions, a constant power supply is necessary.
There is no simple recommendation for building a conventional robot because its characteristics are governed by its application. In the case of small-scale robotics, the need for the problem to be solved will determine the scale of the robot, its range of motion and force, the types of sensors, and compatibility with the environment, as well as its manufacturing process.
Milibots can incorporate control elements, sensors, actuators, and power supply through microfabrication techniques. Some millibot designs use the physiognomy of insects, to achieve their ability to lift several times their weight, build structures (ants), jump with accelerations above 400 g (grasshoppers), or have high sensitivity in flight to deliver a payload (bees in the pollination process).
One of the most representative millibots is RoboBee, designed by Harvard University, which is based on the configuration of a bee with potential applications in agriculture, surveillance, search, and rescue. RoboBee is about half the size of a paper clip, weighs less than one-tenth of a gram, and flies using "artificial muscles" composed of materials that contract when a voltage is applied. A challenge in developing such robotic insects is obtaining electrical power from the environment using piezoelectric, triboelectric, flexoelectric, or hybrid materials.
Microbots are smaller than millibots and their designs are similar to those of some microorganisms. The incorporation of batteries in microbots is complicated and therefore they are powered by external sources. The integration of sensing and control capabilities in microbots are areas of research opportunity.
Nanobots are sub-micrometer scale devices that can use building blocks of biological origin, such as DNA. Nanobots can move through a fluid, but experience drag forces that hinder their propulsion and control. The movement of these nanobots depends on the random motion of the surrounding fluid. Examples of these nanobots are nanoparticle systems for chemotherapy drug delivery, which can target cancer cells and release the drug.
Other types of nanobots use directed chemical actuators, with controlled propulsion capabilities. Rouville et al. (2018) developed nanovehicles for the First Nanocar Race held in April 2017, in which they considered a model of a nanocar with a flat chassis and an optimized nanocar design with a curved chassis.
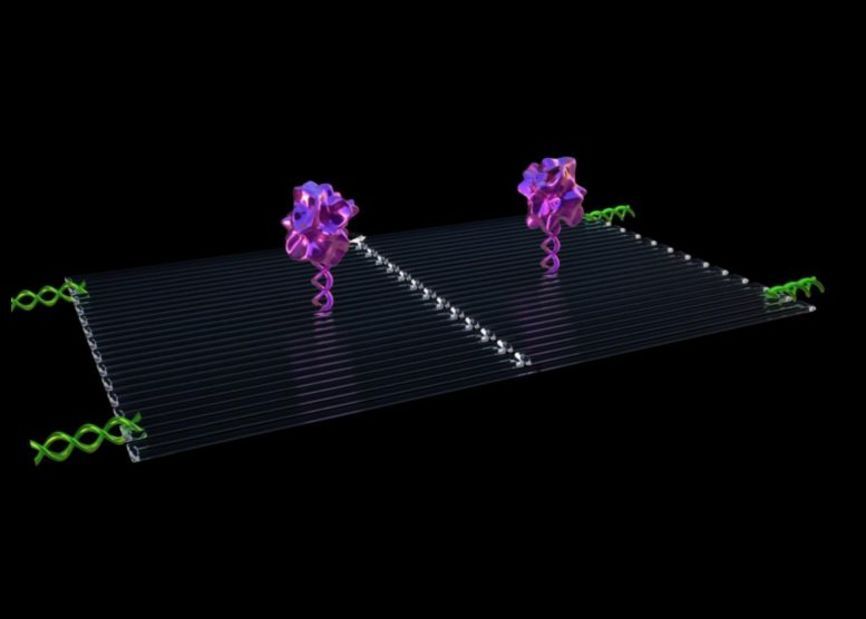
Materials for small-scale robotics
The choice of material depends on the application and size of the robot, although the criteria for its choice may be the desired force, range of motion, manufacturing costs, or compatibility with the environment. Piezoelectric materials are an option due to their property to deform upon application of a voltage. Silicon is another suitable material for microbots when using microfabrication techniques such as MEMS.
For example, in MEMS technology, high-precision electrostatic micropins based on silicon have been developed. In addition, polymers can be used for the implementation of actuators for small-scale robots. However, when considering the operation of a small-scale robot inside the human body, biological compatibility and toxicity become major constraints.
Control of small-scale robotics
In every robotic device, the control stage is responsible for maintaining the device in the desired state. This stage compares the current state with the desired state, and if it detects a deviation, the controller will issue a correction signal to the robot's actuators. Thus, the difference between the current state and the desired state will be eliminated, keeping the robot in the designed behavior.
Milibots can have integrated control elements that operate reactively; however, designs involving swarm-like use of miniaturized robots have also been explored. These devices exhibit limited intelligence but are capable of interacting by exhibiting more complex patterns of behavior, mimicking the behavior of insects such as ants and bees.
Generally, microbots are controlled by devices external to their structures, such as coils, in charge of controlling their trajectory. Several microbots could be controlled using Response Selective Control Global (RSCG), which designs the trajectories of the microbots. Control of nanobots within a fluid can be obtained by randomly moving their surrounding fluid for propulsion, or using motor proteins, such as kinesin, which allows moving nanometer systems through microtubules or finding the entry point of a cell.

The power supply of small-scale robotics
The implementation of the power supply in a small-scale robot is related to its size and behavioral specifications. For example, millibots can have a power supply integrated, using piezoelectric materials, thermal memory effect alloys, or polymers. There are several options for power supply in microbots operating in a liquid environment. These microbots can be driven by remotely generated forces, such as those obtained with the use of external magnetic coils, or self-propulsion methods using biomaterials such as cells or bacteria.
The conversion of energy from the environment into electrical energy using piezoelectric, triboelectric, or flexoelectric materials is an alternative for supplying power to small-scale robots. The concept involves the conversion of environmental energy to power small electrical and electronic devices to make them self-sufficient. For example, a millibot can convert mechanical energy from environmental vibrations into electrical energy. A small-scale robot operating in the human body could power its energy cells using the patient's natural temperature, and so on.
Applications of small-scale robotics
Applications for millibots include scientific instrumentation, manufacturing, and medicine. Robots on this scale can be used to study new materials or biological samples. Millibots can be employed to manufacture a large number of small mobile robots, fulfilling one of Feynman's envisioned goals for these technologies. In medicine, these robots can be like a camera encapsulated in a pill, which can take samples of objects such as a tumor, or other abnormalities in the human body. There are robots on this scale capable of manipulating and repairing the intestinal tract.
Microbots have applications in medicine and can function as tools for noninvasive surgery, guided drug delivery, and tissue engineering. These types of robots could be used to realize the "factory-on-chip" concept, in which they could participate in the assembly of complex microstructures. These microbots could also perform maintenance work for complex microstructures and repair them. Other applications could include surveillance and information security, where the size of these machines makes them almost undetectable.
Nanobots are an alternative for the treatment of different types of cancer, considering nanoparticle systems capable of delivering drugs to specific parts of the human body, without affecting healthy cells. Other nanobot systems made of DNA components could repair damaged chromosomes. Finally, robotic systems could be used to remove contaminants from water.
Source: Elementos, Authors: Gerardo Mora Aquino (University of Valle de México) and Agustín Leobardo Herrera May (Center for Research in Micro and Nanotechnology)